
Life Science/Biology
Engineering Connection: Organ Donation (Chapter 8, p. 1040)
Students can learn about the role of engineering to meet critical medical needs to solve another problem in organ donation: matching suitable donors with patients.
In addition to striking examples of engineering like magnetic resonance imaging (MRI) and robotic surgery, some engineers also develop important processes such as, matching donors and patients by breaking down the problem into smaller, more manageable problems. Students can consider the different aspects of the problem of donor matching (e.g. awareness about the process by potential donors, rapid and reliable genetic testing, etc.) and brainstorm and evaluate possible solutions to them.
Engineering Connection: Wastewater Treatment Facilities (Chapter 8, p. 1052)
When raw sewage flows into waterways, it can impact the health of both humans and ecosystems (EP&Cs II, IV), which is why wastewater treatment facilities are an important part of all California cities. Engineers have learned to put biological processes to work to process human waste in wastewater treatment facilities. Students can obtain information (SEP-8) about the different stages of sewage treatment, some of which involve bacteria that rapidly decompose organic waste. Students can make physical models (SEP-2) of this process by using sugars to represent the organic waste, yeast to represent the waste-processing bacteria, and glucose test strips to measure the concentration of simulated waste in the water. Performing investigations using these models, students can develop techniques for speeding up the wastewater treatment process. Is there an optimal amount of yeast to add? Does the treatment process speed up or slow down when students add air or seal the container? What techniques can they develop for efficiently adding air?
Engineering Connection -Life Science-Biology (Chapter 8, p. 1057)
Within ecosystem science, some aspects of conservation biology are a form of engineering that focuses on developing processes that save endangered and threatened species (both animal and plant species). Conservation biologists help preserve these species by: (1) supporting the use of wildlife corridors, which link large areas of land to other large areas so animals can migrate safely; (2) developing breeding programs for protecting endangered species; (3) identifying specific hotspots of species-rich regions worthy of extra protection and determining plans that provide sufficient protection; (4) arguing for the maintenance of larger environment regions instead of habitat fragmentation; (5) observing genetic diversity in small populations; and (6) monitoring the effects of climate change on all ecosystems. Students can investigate one specific environmental change that threatens biodiversity and propose a solution (SEP-8). As they obtain more information (SEP-8), including the needs of people, as well as, plants and other animals, they refine their solution (EP&C V; HS-LS2-7).
Engineering Connection – Life Science-Biology (p. 1082)
When humans sprayed the pesticide DDT on farm fields in California, it accumulated in animal tissues and affected the viability of many bird eggs, including eggs of the California condor. The condor populations plummeted until the pesticide was banned in 1972 due to concerns over its impact on bird populations (EP&C II, IV).
By that point, the condor population had dwindled so far that only a few dozen birds remained. Students can develop or revise a computer simulation (HS-LS4-6) of a condor captive breeding and release program like the one implemented in California (Ventana Wildlife Society n.d. ). How quickly can the population expect to recover? How many breeding pairs should be captured initially to ensure a diverse enough gene pool? Students can track the variation in traits of offspring from one generation to the next and use that to estimate the susceptibility to selective pressures such as disease or drought.
Engineering Connection: Chemistry (p. 1150)
The Chemistry of Global Energy Supplies
A key aspect of three-dimensional learning is that students use SEPs to apply CCCs to DCIs in other fields of study. The flows, cycles, and conservation of energy and matter (CCC-5)l is one of the most unifying crosscutting concepts, providing links between all of the sciences and many other fields of study as well, including the social and behavioral sciences. Figure 8.26, illustrates the growth of the world population during the last millennium, including a forecast for the twenty-first century. Students should be able to read such a graph and recognize that there will be twice as many people by 2100, as there were in 2000. Consequently, there will be an increased demand for all resources (EP&C I), but particularly energy resources.
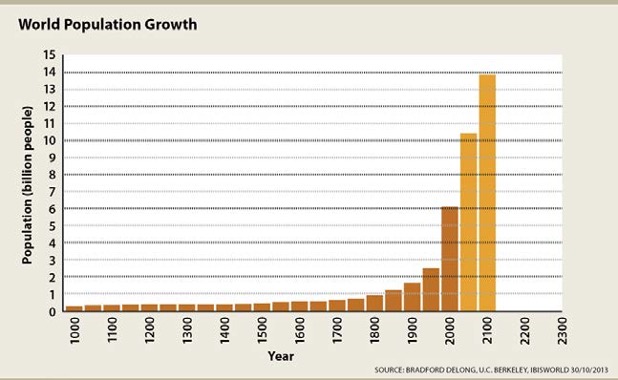
Figure 8.27 shows trends in world energy consumption and illustrates that the three major sources of energy worldwide are fossil fuels (oil, coal, and natural gas). Students can obtain information about the impacts of fossil fuels on natural systems that arise because harnessing the energy from fossil fuels also disrupts global cycles of matter in the Earth system (ESS2 A; EP&C III, IV). Climate change results from rising levels of greenhouse gases (e.g., carbon dioxide, methane, and nitrous oxide). Carbon dioxide is released when fossil fuels react with oxygen during combustion, and students can obtain more information (SEP-8) about chemical methods of carbon sequestration currently being researched. Natural gas, primarily methane, often leaks at many locations between where it is pumped from the ground and where people eventually use it. Students can obtain more information (SEP-8) about cutting-edge technologies to monitor leaks in real time.
Engineering Connection: Chemistry (p. 1151)
The Chemistry of Global Energy Supplies
Acid rain results from nitrogen and sulfur oxides commonly released during combustion of sulfur-rich fuels such as coal. Students could obtain more information (SEP-8) about the chemical technology used to minimize the release of sulfur dioxide. Since these systems were mandated, acid rain has substantially declined in the United States. Smog involves reactions between tailpipe emissions of cars and the air (with sunlight adding some of the energy to break chemical bonds). Students could also obtain more information (SEP-8) about how improvements to the combustion efficiency of cars have reduced smog. Students should do more than just explain the chemical reactions in each of these processes. They should consider the criteria and constraints about society’s need for clean air and clean water along with the need for more energy (HS-ETS1-1; EP&C V). Students should be encouraged to break down the problem into smaller, more manageable problems that can be solved through [chemical] engineering (HS-ETS1-2).
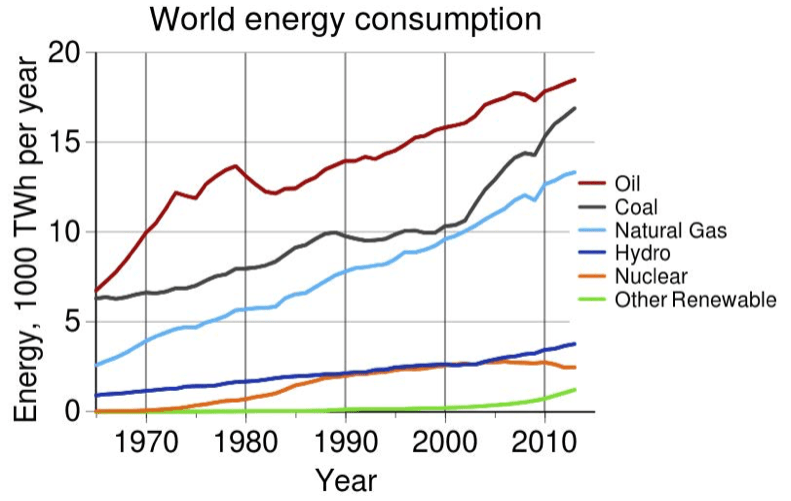
Engineering Connection: Chemistry
Designing an Energy Conversion Device (p. 1152)
Engineers have designed a range of devices to convert chemical potential energy in organic matter to other forms of energy. Cars, gas furnaces, and wood stoves are all examples. Students can build their own device to convert energy from one form to another by designing (SEP-6) calorimeters that combust food to transform chemical potential energy into light energy and thermal energy (CCC-5) (HS-PS3-3, HS-ETS1-2). By using a calorimeter to measure the caloric density of various foods, students can calculate (SEP-5) the approximate amount (CCC-3) of chemical potential energy in such things as jellybeans, cashews, oily snack foods, and more. They can then refine their design to convert the greatest percentage of chemical potential energy from these foods into heat energy during combustion of the food so that it provides the most reliable estimates of food energy (HS-ETS1-3). The efficiency of such calorimeters may be estimated by dividing the thermal energy captured in the water of calorimeters by the calculated caloric value present in the food prior to burning. Regardless of the selected activities, students should apply scientific and engineering reasoning to define problems (SEP-1) and design and optimize solutions (SEP-6) to real-world devices that convert chemical potential energy into other energy forms.
Engineering Connection: Physics
Minimizing the Effects of Collisions (p. 1165)
Equipped with a basic understanding of classical mechanics, including Newton’s three laws of motion and the momentum conservation principle, students should now be able to “apply scientific and engineering ideas to design, evaluate, and refine a device that minimizes the force on a macroscopic object during a collision” (HS-PS2-3). A classic activity that meets this performance expectation is the egg-drop contest, in which students are challenged to develop devices that protect raw eggs from breaking when dropped from significant heights (figure 8 30). In the process, students demonstrate competence with HS-ETS1-1 when they start by considering a complex problem such as automobile collisions or sports injuries, and then they define the problem (SEP-1) in terms of qualitative and quantitative criteria and constraints for solutions. With teacher guidance, the students can then break down the problem into smaller, more manageable problems that can be solved through engineering (HS- ETS1-2). The students should be encouraged to generate multiple solutions (SEP-6), and to evaluate their ideas based on prioritized criteria and trade-offs (see the section on Decision Matrices in the “Instructional Strategies” chapter of this framework), taking into account cost, safety, and reliability as well as social, cultural, and environmental impacts (HS-ETS1-3). Students then build and test a model of their most promising idea and then modify it based on the results of the tests. Testing can include computer simulations that model how solutions function under different conditions (HS-ETS1-4)
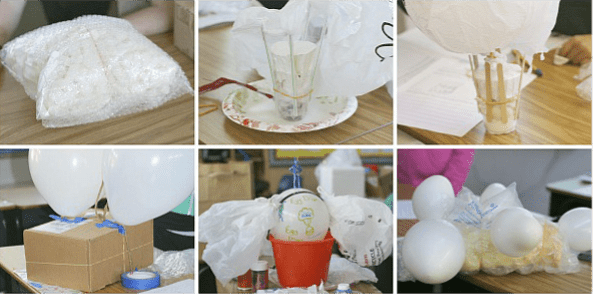
Students learn physics principles such as impulse and momentum while simultaneously learning engineering design and testing principles, while designing and developing devices for challenges, such as, the classic egg-drop contest. Source: Buggy and Buddy 2014 Long description of Figure 8.30
Engineering Connection: Physics
Minimizing the Effects of Collisions (p. 1166)
Throughout the process, students should justify (SEP-7) their design choices and revisions in terms of physics concepts, rather than using trial and error or guesswork. The engineering solutions students create are examples of systems (CCC-4) of interacting components. Students discover that the exact physical structure (CCC-6) (the arrangement of the components) can have a large impact on the function of their design. Students can draw pictorial models (SEP-2) showing the direction forces act and can label the role each piece plays in their solution.
Engagement in this activity also tests student understanding of the momentum-impulse connection: FΔt = mΔv, where F = force, t = time, m = mass, and v = velocity. The product of force and the time over which the force is applied is known as the impulse (FΔt) and is equal to the change in momentum of the object to which the force is applied (mΔv). One can decrease the force necessary to bring a moving object to rest by increasing the time over which the force is applied. For example, air bags, car crumple zones, helmets, parachutes, and padded catcher’s mitts (figure 8 31) reduce the potential for injury by decreasing the force necessary to bring objects to a halt by increasing the time over, which such forces are applied.
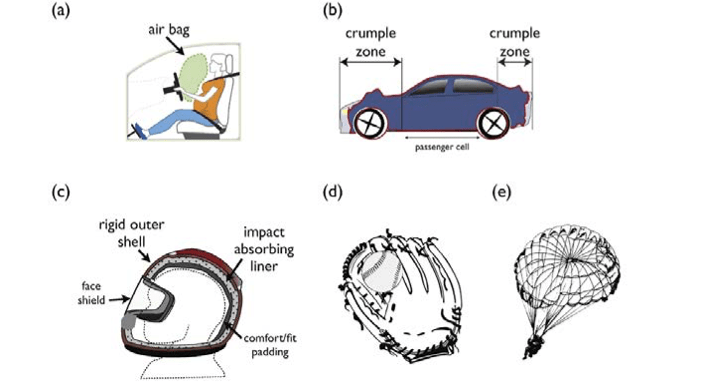
Long description of Figure 8.31
The product of force and the time over which the force is applied is known as the impulse (FΔt), and is equal to the change in momentum of the object to which the force is applied (mΔv). One can decrease the force (F) necessary to bring a moving object to rest by increasing the time (Δt) over which the force is applied, such as is accomplished by (a) an automobile air bag, (b) a helmet, (c) a baseball catcher’s mitt, or (d) a parachute Sources: M d’Alessio with images from National Highway Traffic Safety Administration 2015; adapted from KTEditor 2014; adapted from Open Clipart-Vectors 2013b; Clker-Free-Vector-Images 2012; Open Clipart-Vectors 2013a; Headquarters Department of the Army 2012
Engineering Connection: Physics
Build an Energy Conversion Device (p. 1197)
Humans are natural engineers. We learn to modify our environment to solve specific problems, as seen when a child moves a chair to access the cookie jar or when a young person designs props for the school play. Engineering skills can be developed while studying science as students define and design solutions to real-world problems in chemistry, physics, biology, and the Earth and space sciences.
Science students can demonstrate competence in all three phases of the engineering process: defining problems (SEP-1) defining solutions (SEP-6) to these problems, and optimizing design solutions HS-PS3-3 requires students to “design, build, and refine a device that works within given constraints to convert one form of energy into another form of energy”. Equipped with a basic understanding of the laws of thermodynamics, students understand that energy can be converted from less useful forms to more useful forms. This performance expectation challenges students to design and build devices, such as wind turbines, solar ovens, or electric generators to transform energy from less useful forms, such as wind, sunlight, or motion, into electricity, the most convenient and useful form of energy in our modern world. Students deepen their understanding of the core ideas of science as they increase their competence in engineering design through such activities. Students should first consider the major global challenge of providing affordable electrical energy (HS- ETS1-1). Designing, building, and improving energy conversion devices that are more efficient or that pollute less involves breaking down the complex global problem into more manageable problems that can be solved through engineering (HS-ETS1-2). In the laboratory, students learn to work within engineering constraints as they strive to maximize efficiency (minimize energy loss), while designing and building devices with limited resources in a limited timeframe. Students can measure outputs and then refine their designs to maximize efficiency given constant inputs. As they make decisions, students need to keep in mind prioritized criteria and trade-offs (HS-ETS1-3). As a follow-up to the laboratory, students can explore the acceptability of different solutions to the global energy problem, taking into account social, cultural, and environmental impacts (HS-ETS1-3). Students can also use existing computer simulations to investigate the impact of different energy solutions (HS-ETS1-4).
Engineering Connection: Physics
Using Waves to Transmit Information (p. 1206)
Performance expectation HS-PS4-5 is closely tied to the ETS standard HS-ETS1-2: “Design a solution to a complex real-world problem by breaking it down into smaller, more manageable problems that can be solved through engineering”. Students may meet both the physical science and the ETS performance expectations by designing their own devices where electromagnetic waves interact with matter to transmit and capture information.
Engineering Connection: Earth and Space Sciences
Evaluating Renewable Energy Options (p. 1233)
Ultimately, any discussion of climate change should begin to explore technological solutions that could reduce emissions of greenhouse gases. In a classroom, students can calculate their own carbon footprints to further understand how they contribute to the human impacts on the global carbon cycle. They can explore renewable energy options and debate the pros and cons of each possible energy source for meeting society’s needs (National Energy Education Development Project 2012b) (HS-ESS3-2, HS-ETS1-1). They can complete the project by creating another summary product for their school that communicates (SEP-8) some steps that individuals could take to reduce their impact on the climate system, or recommend broader actions that their school and community could take that will have an even larger effect.
Engineering Connection: Earth and Space Sciences
Mitigating Erosion Hazards (p. 1241)
When the natural process of erosion affects humans, it becomes a natural hazard. Students can explain some of the common impacts of erosion in California (HS- ESS3-1). They can also engage in an engineering design problem to reduce these impacts (HS-ESS3-4). Students can design and build erosion control measures using stream tables, as well as, read about actual measures that are taken in places like Pacifica and locations all along the California coastline. The engineering solutions either involve: (1) increasing the strength of the hillside (by adding plants with root systems to stabilize the hillside, building support walls, or covering the cliff with concrete); or (2) reducing the driving forces (by placing rocks or sea walls to reduce the speed of waves when they hit the natural hillslope and through better drainage). Students should compare and evaluate solutions based on prioritized criteria and tradeoffs that account for a range of constraints, including cost, safety, reliability, and aesthetics (HS-ETS1-3; EP&C V). Sometimes, technologies that reduce the impact of erosion on people can have adverse impacts on ecosystems (EP&C III). Students should consider and evaluate the environmental impacts of their design and refine it to reduce those impacts (HS-ESS3-4).
Engineering Connection: Earth and Space Sciences
Water Filtration (p. 1248)
California is home to state-of-the art water treatment plants and rigorously enforced regulations, but much of the rest of the world struggles to find clean and safe water. Students could obtain information (SEP-8) about water treatment and purification systems in their community, or be given engineering challenges to design water purification systems (such as Engineering is Elementary, Water, Water Everywhere at https://www.cde.ca.gov/ci/sc/cf/ch8 asp#link61). These systems have a lot in common with the natural groundwater system, which is an excellent filtration system. This is why digging groundwater wells can dramatically decrease health risks from waterborne pathogens in third-world countries (HS-ETS1-1). This framework calls on students to perform a similar design process in fifth grade, but students can return to the problem in high school with a broader understanding of the properties of water.
Engineering Connection: Reducing Urban Runoff (p. 1273)
One of the biggest changes (CCC-7) an urban landscape makes upon the world is covering soil with impermeable concrete and structures. These changes (CCC-7) disrupt the natural hydrologic cycle, preventing water from soaking in and becoming groundwater and instead sending it into river channels where it can cause flooding, increased erosion, or both. As water moves across the surface in the built environment, it carries contaminants into these waterways contaminating the water. Students can apply their knowledge of Earth materials to explore solutions to the urban runoff problem by designing systems to catch and filter runoff before it enters waterways (see Engineering is Elementary Don’t Runoff at https://www.cde.ca.gov/ci/ sc/cf/ch8 asp#link86) (HS-ESS3-4; HS-ETS1-2). Harmful pollutants are just one of the many ways that urban areas have significant impact on plant and animal life in a region (anthrosphere-biosphere interactions, LS4 D; EP&C II).
Engineering Connection: Computational Models of Orbit (p. 1293)
When a company spends millions of dollars to launch a communications satellite or the government launches a new weather satellite, they employ computer models of orbital motion to make sure these satellites will stay in orbit and the investment is not lost. These models (SEP-5) are based on the exact equations introduced in the CA NGSS high school courses. In fact, students can gain a deeper understanding of the orbital relationships and develop computational thinking (SEP-5) skills by interacting directly with computer models of simple two-body systems (CCC-4). Even with minimal computer programming background, students could learn to interpret an existing computer program of a two-body gravitational system. They could start by being challenged to identify an error in the implementation of the gravity equations in sample code given to them. Next, students modify the code to correctly reflect the mass of the Earth and a small artificial communications satellite orbiting around it. They can vary different parameters in the code such as the distance from Earth or initial speed and see how those parameters affect the path of the satellite (HS-ESS1-4). At what initial launch speeds will the satellite stay in orbit? What is the tradeoff between the cost of fuel and the payload mass?
(Note: Appendix 3 in this framework provides guidance about teaching computer coding aligned with the CA NGSS.)