
The Living Earth -Integrating Biology and Earth Science
Engineering Connection: Wastewater Treatment Facilities (Chpt. 7, p. 794)
When raw sewage flows into waterways, it can impact the health of both humans and ecosystems (EP&Cs II, IV), which is why wastewater treatment facilities are an important part of all California cities. Engineers have learned to put biological processes to work to process human waste in wastewater treatment facilities. Students can obtain information (SEP-8) about the different stages of sewage treatment, some of which involve bacteria that rapidly decompose organic waste. Students can make physical models (SEP-2) of this process by using sugars to represent the organic waste, yeast to represent the waste-processing bacteria, and glucose test strips to measure the concentration of simulated waste in the water. Performing investigations using these models, students can develop techniques for speeding up the wastewater treatment process. Is there an optimal amount of yeast to add? Does the treatment process speed up or slow down when students add air or seal the container? What techniques can they develop for efficiently adding air? Students can construct an explanation (SEP-6) about how the change in oxygen in the bacteria’s environment affects their respiration rate (HS-LS2-3).
Engineering Connection: Coastline Erosion (Chapter 7, p. 806)
When coastline erosion affects humans, it becomes a natural hazard. Students can explain some of the common impacts of erosion in California. (HS-ESS3-1) They can also engage in an engineering design problem to reduce these impacts. (HS- ESS3-4) Students can design and build erosion control measures using stream tables, as well as, read about actual measures that are taken in places like Pacifica and locations all along the California coastline. The engineering solutions either involve (1) increasing the strength of the hillside (by adding plants with root systems to stabilize the hillside, building support walls, or covering the cliff with concrete); or (2) reducing the driving forces (by placing rocks or sea walls to reduce the speed of waves when they hit the natural hillslope and through better drainage). Students should compare and evaluate solutions based on prioritized criteria and tradeoffs that account for a range of constraints, including cost, safety, reliability, and aesthetics. (HS-ETS1-3; EP&C V) Sometimes, technologies that reduce the impact of erosion on people can have adverse impacts on ecosystems. (EP&C III) Students should consider and evaluate the environmental impacts of their design and refine it to reduce those impacts. (HS- ESS3-4) Students can obtain information (SEP-8) about different California coastal communities and explain (SEP-6) why they have chosen to develop or not develop their coastlines. (HS-ESS3-1)
Engineering Connection: Organ Donation (Chapter 7, p. 827)
Students can learn about the role of engineering to meet critical medical needs to solve another problem in organ donation: matching suitable donors with patients. In addition to striking examples of engineering like magnetic resonance imaging (MRI) and robotic surgery, some engineers also develop important processes such as matching donors and patients by breaking down the problem into smaller, more manageable problems. Students can consider the different aspects of the problem of donor matching (e.g. awareness about the process by potential donors, rapid and reliable genetic testing, etc.) and brainstorm and evaluate possible solutions to them.
Engineering Connection: Conservation Biology (Chapter 7, p. 839)
When conservation biologists develop strategies to save endangered and threatened species, they are engaging in one form of engineering design. Conservation biologists help save these species by (1) supporting the use of wildlife corridors, which link large areas of land to other large areas so animals can migrate safely; (2) developing breeding programs for protecting endangered species; (3) identifying specific hotspots of species-rich regions worthy of extra protection and determining plans that provide sufficient protection; (4) arguing for the maintenance of larger environment regions instead of habitat fragmentation; (5) observing genetic diversity in small populations; and (6) monitoring the effects of climate change on all ecosystems.
As climate shifts, some organisms might need to migrate to new locations during part or all of the year, but their pathways could be interrupted by a freeway, fence, or other obstacles. Teachers can present students with a challenge to evaluate several possible plans for a wildlife corridor beneath a freeway and the possible expansion of a protected open space, which would allow them to use engineering design practices to solve a real-world problem (SEP-6) in an ecosystem using the tools and strategies of conservation biology. As they obtain more information (SEP-8), including the needs of people, as well as plants and other animals, they refine their solution. (EP&C V; HS-LS2-7)
Chemistry in the Earth System
Engineering Connection:
The Chemistry of Global Energy Supplies (Chapter 7, p. 908)
Figure 7.37 graphs trends in world energy consumption and illustrates that the three major sources of energy worldwide are fossil fuels (oil, coal, and natural gas). Students can obtain information about the impacts of fossil fuels on natural systems that arise because harnessing the energy from fossil fuels also disrupts global cycles of matter in the Earth system. (ESS2 A; EP&C III, IV) Climate change results from rising levels of greenhouse gases (e.g., carbon dioxide, methane, and nitrous oxide). Carbon dioxide is released when fossil fuels react with oxygen during combustion, and students can obtain information (SEP-8) about chemical methods of carbon sequestration that are current research topics. Natural gas is primarily methane, which can leak into the atmosphere during production, processing, transport, storage, and distribution. Students can obtain information (SEP-8) about cutting-edge technologies to monitor leaks in real time. Acid rain results from nitrogen and sulfur oxides commonly released during combustion of sulfur rich fuels such as coal. Students could obtain information (SEP-8) about the chemical technology used to minimize the release of sulfur dioxide.
Since these systems were mandated, acid rain has substantially declined in the United States Smog involves reactions between tailpipe emissions of cars and the air (with sunlight adding some of the energy to break chemical bonds). Students could also obtain information (SEP-8) about how improvements to the combustion efficiency of cars have reduced smog. Students should do more than just explain the chemical reactions in each of these processes. They should consider the criteria and constraints about society’s need for clean air and clean water along with the need for more energy (HS-ETS1-1; EP&C V). Students should be encouraged to break down the problem into smaller, more manageable problems that can be solved through [chemical] engineering. (HS-ETS1-2)
Engineering Connection: Testing Material Strength (Chpt. 7, p. 935)
Newton’s second law can also be used to test the strength of different materials for a design challenge. A satellite must withstand vibrations from a rocket launch, a hospital must withstand earthquake shaking, and a child’s toy must be able to withstand being sat on by a toddler. In many of these cases, it is not practical to do iterative testing on the actual objects (they cannot build various trials of a hospital and have each of them fall down—each one takes years and cost millions of dollars to complete). Instead, engineers do calculations to test their designs before investing the time and materials to actually build a prototype. In the classroom, students could determine the maximum force a toothpick can withstand before it snaps or a toilet paper tube before it buckles. They do this by placing heavy objects on top of the test material and measuring the amount of mass that causes (CCC-2) the material to break.
Since the acceleration of gravity is constant, the force can be calculated using the mathematical model W = mg (a special case of F = ma where W is the force of the object’s weight, m is mass, and g is the constant of gravitational acceleration). By comparing this force to calculations of the expected force on impact during a design challenge, they can make informed decisions about materials. Engineers perform similar calculations to provide evidence that their design will withstand the expected forces. They often use computer simulations like in figure 7.44 to perform these calculations.
Engineering Connection: Collision Challenge (Chapter 7, p. 940)
Equipped with a basic understanding of classical mechanics, including Newton’s three laws of motion and the momentum conservation principle, students should now be able to “apply scientific and engineering ideas to design, evaluate, and refine a device that minimizes the force on a macroscopic object during a collision” (HS- PS2-3).
A classic activity that meets this performance expectation is the egg-drop contest, in which students are challenged to develop devices that protect raw eggs from breaking when dropped from significant heights (figure 7.48). In the process, students demonstrate competence with HS-ETS1-1; they start by considering a complex problem such as automobile collisions or sports injuries, and then they defend the problem (SEP-1) in terms of qualitative and quantitative criteria and constraints for solutions. With teacher guidance the students can then divide the entire problem into smaller, more manageable problems that can be solved through engineering (HS- ETS1-2). The students should be encouraged to generate multiple solutions (SEP-6), and to evaluate their ideas based on prioritized criteria and trade-offs (see the section on decision matrices in chapter 11 of this framework), taking into account cost, safety, and reliability as well as social, cultural, and environmental impacts (HS-ETS1-3). Students then build and test a model of their most promising idea and modify it based on the results of the tests. Testing can include computer simulations that model how solutions function under different conditions. (HS-ETS1-4)
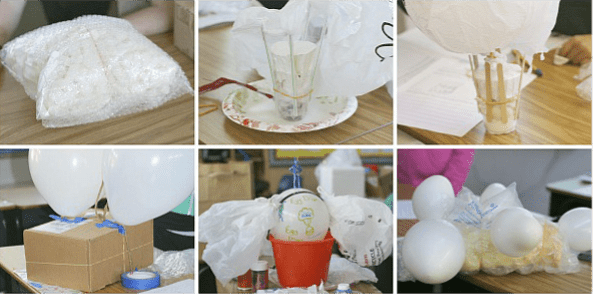
Students learn physics principles such as impulse and momentum while simultaneously learning engineering design and testing principles while designing and developing devices for challenges such as the classic egg-drop contest Source: Buggy and Buddy 2014 Long description of Figure 7.
Engineering Connection: Computational Models of Orbit (p. 949)
When a company spends millions of dollars to launch a communications satellite or the government launches a new weather satellite, they employ computer models of orbital motion to make sure these satellites will stay in orbit and the investment is not lost. These models (SEP-2) are based on the exact equations introduced in the CA NGSS high school courses. In fact, students can gain a deeper understanding of the orbital relationships and develop computational thinking (SEP-5) skills by interacting directly with computer models of simple two-body systems (CCC-4). Even with minimal computer programming background, students could learn to interpret an existing computer program of a two-body gravitational system. They could start by being challenged to identify an error in the implementation of the gravity equations in sample code given to them. Next, students modify the code to correctly reflect the mass of the Earth and a small artificial communications satellite orbiting around it. They can vary different parameters in the code such as the distance from Earth or initial speed and see how those parameters affect the path of the satellite (HS-ESS1-4). At what initial launch speeds will the satellite stay in orbit? What is the tradeoff between the cost of fuel and the payload mass? (Note: Appendix 3 in this framework provides guidance about teaching computer coding aligned with the CA NGSS.)
Engineering Connection:
Engineering Energy Conversion Devices (Chapter 7, p. 963)
Now that students have learned extensively about the theory behind energy conversion (CCC-5) devices, they are now tasked with an engineering challenge to create one themselves. (HS-PS3-3) The vignette in the High School Four-Course Model—Physics course (chapter 8) in this framework includes a template of what this design challenge might look like. The first stage of the engineering design process is to place the goal in the context of the major global challenge of providing affordable electrical energy without the problems associated with fossil fuels. (HS-ETS1-1) Students evaluated the impacts of different electricity sources at the beginning of this instructional segment, including a discussion of how fossil fuels contribute to global climate change.
The High School Three-Course Model—Chemistry in the Earth System course emphasizes physical mechanisms causing climate change and the High School Three-Course Model—The Living Earth course explores its effects on the biosphere. Depending on the sequence of courses within each school district, this instructional segment should draw strong connections to those courses. Designing, building, and improving energy conversion devices that are more efficient or that pollute less involves breaking down the complex global problem into more manageable problems that can be solved through engineering. (HS-ETS1-2)
Students have learned some of the scientific principles behind the engineering tools that can help address the challenge throughout this instructional segment. Students now choose to build their own wind turbines, hydroelectric power plants, solar panels, or other mini-version of a power plant that transforms energy from less useful forms, such as wind, sunlight, or motion, into electricity (arguably the most convenient and useful form of energy in our modern world). Students learn to work within engineering constraints as they strive to maximize efficiency (generate the largest power output possible) while taking into account prioritized criteria and trade-offs. (HS-ETS1-3) Students can measure outputs and then refine their designs to maximize efficiency given constant inputs Students can also use existing computer simulations to investigate the impact of these different energy solutions (HS-ETS1-4).